Workshops & Meetings
RATIC Workshop
ASSW 2019 in Arkhangelsk, Russia | 26 May
The RATIC 2019 workshop was held on 26 May 2019 at the NArFU Intellectual Center in Arkhangelsk, Russia, in conjunction with IASC's Arctic Science Summit Week. Workshop participants were asked to come with thoughts on where they can most effectively plug into RATIC, matching their interest or expertise to this cross-cutting topic. During the workshop, over 50 participants identified the most pressing research questions related to impacts of climate and infrastructure in the natural environment (terrestrial/ecological/cryopshere), social environment (social/human dimensions), and built environment (engineering/industry), and then discussed new ways to connect them. At the end of this full-day meeting, the group explored how to continue to promote sustainable Arctic infrastructure and international multidisciplinary collaboration as a T-MOSAiC Arctic Infrastructure Action Group. There was no cost to attend the workshop, which was sponsored by the International Arctic Science Committee.
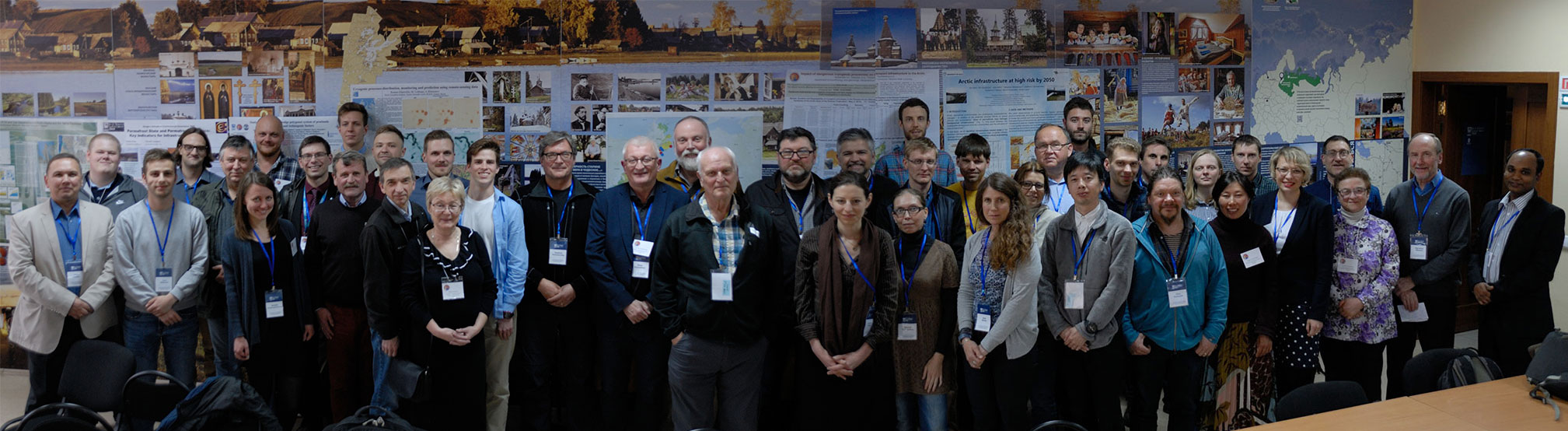
Oral Presentations
Session 1: Impacts and interactions of climate and infrastructure in the Arctic
- Intro and Next Steps | Walker & Peirce, USA (Presentation 12.2 MB)
- Response of permafrost environment to natural changes and human impact in the north
of West Siberia (Yamal-Nenets Autonomous Okrug) | Leibman, Russia (Presentation 41.6 MB, Abstract) - Modeling of circumpolar permafrost and permafrost-thaw related geohazards affecting infrastructure | Karjalainen, Finland (Presentation 3.8 MB, Abstract)
- Impacts of climate change and infrastructure on reindeer herding in the Yamal peninsula | Kumpula & Laptander, Finland (Abstract)
Session 2: Approaches to research and adaptation
- T-MOSAiC and RATIC: Connections and opportunities | Vincent, Canada (Presentation 2.6 MB, Abstract)
- Navigating the new Arctic: Landscape evolution and adapting to change in ice-rich-permafrost systems (NNA-IRPS) | Walker, USA (Presentation 65.1 MB, Abstract)
- Sustainable housing in rural Alaska | Garber-Slaght, USA (Presentation 7.8 MB, Abstract)
- Infrastructure stability estimation: Usage of GTN-P data and permafrost forecasting | Sergeev & Utkina, Russia (Presentation 25 MB, Abstract)
- Social science perspectives on Arctic infrastructure | Schweitzer & Povoroznyuk, Austria (Presentation 23.7 MB, Abstract)
- Prague workshop synthesis | Peirce, USA (Presentation 6.3 MB)
Poster Session
- Arctic infrastructure at high risk by 2050 | Hjort et al. (Poster 9.9 MB, Abstract)
- Assessment of nutrient concentration and availability in ice-wedge polygon successional stages in a coastal Arctic tussock tundra in Jago, Alaska | Kent et al. (Poster 16.5 MB, Abstract)
- Configurations of “remoteness” (CoRe) - Entanglements of Humans and Transportation Infrastructure in the Baykal-Amur Mainline (BAM) Region | Schweitzer et al. (Poster 7.9 MB, Abstract)
- Cryogenic processes distribution, monitoring and prediction using remote-sensing data | Khairullin (Poster 22.2 MB, Abstract)
- Dynamics of active layer depth and ground temperature under climatic fluctuations and technogenic impact | Babkina et al. (Abstract)
- Influence of the climatic factor on the mechanical properties of frozen soils (region of the Yuribey river, Yamal Peninsula) | Agapkin et al. (Poster 2.7 MB, Abstract)
- Permafrost State and Permafrost Dynamics: Key Indicators for Infrastructure Stability | Sergeev & Utkina (Poster 6.2 MB, Abstract)
- Study of dynamics of ice wedge polygonal system of peatlands under climatic and technogenic factors | Babkin et al. (Abstract)
- Impact of dangerious cryogenic processes on the transport infrastrucure in the Arctic | Grebenets & Tolmanov (Poster 6.1 MB, Abstract)
- Vegetation along the 1700-km Yamal Peninsula–Franz Josef Land Eurasia Arctic Transect | Walker et al. (Poster 15.2 MB, Abstract)
Abstracts
Session 1: Impacts and interactions of climate and infrastructure in the Arctic
Response of permafrost environment to natural changes and human impact in the north of West Siberia (Yamal-Nenets Autonomous Okrug)
Marina O. Leibman1, Artem V. Khomutov1, Yu A. Dvornikov1, Elena A. Babkina1, Rustam R. Khairullin1, Evgeny M. Babkin1, N. Yu Fakashchuk1
(1) Earth Cryosphere Institute, Tyumen Scientific Centre, SB Russian Academy of Sciences, Tyumen, Russia
Our main goal is studying natural permafrost environments and limited human impact caused by infrastructure development in the area close to gas fields, pipelines, railways, roads and quarries. Years of monitoring active layer, ground temperature and landslide activities at Vaskiny Dachi research station, we extended our studies. Spatially we extended monitoring westward to include gas-emission graters (GEC), and eastward to Tazovsky and Gydan peninsulas, south of, and north of Vaskiny Dachi research station latitude.
Research topics were extended as well. The following have been added to the research: (1) field studies (organic matter fate and hydrochemistry in the lake and GEC lake water; distribution and rate of thermocirque growth; dynamics of GEC width and depth); (2) laboratory studies (ionic and isotope analyses of lake and GEC-lake water; methane concentration in lake and GEC-lake water; dissolved organic matter in the lake water. Remote-sensing studies (processing optical images for monitoring GEC, thermocirques, peat plateaus, mapping tabular ground ice, methane emission in the lakes, predicting natural hazards).
We perform measurements of moisture content in the active layer, geochemistry of active-layer soils, bathymetry of lakes, including GEC lakes, snow survey and geochemistry of snow.Results achieved so far are as follows. Since 2012 air temperature increase dramatically resulting in 20% deeper active layer, 0,5 degree higher ground temperature, activation of thermal denudation, formation of GEC transforming into lakes-successors.
It was revealed that the thaw depth increase in 2012 and 2013 reached the top of tabular ground ice and thermocirques were formed. In 2016, the growth of thermocirques speeded up.
Evidently, the presence of gas accumulations inside the permafrost caused by the increase in average ground temperature predetermined the release of gas formed during the dissociation of gas hydrates, with the formation of gas emission craters.
Human impact resulted in deeper active layer along the vehicle tracks, degradation of peat plateau crossed by the road, fast overgrowing of the quarries thanks to warmer summers.
Olli Karjalainen1, Juha Aalto2,3, Miska Luoto2, Sebastian Westermann4, Vladimir E. Romanovsky5,6, Frederick E. Nelson7,8, Bernd Etzelmüller4 & Jan Hjort1
(1) University of Oulu, Geography Research Unit, Oulu, Finland
(2) University of Helsinki, Department of Geosciences and Geography, Helsinki, Finland
(3) Finnish Meteorological Institute, Helsinki, Finland
(4) University of Oslo, Department of Geosciences, Oslo, Norway
(5) University of Alaska Fairbanks, Geophysical Institute, Fairbanks, Alaska, USA
(6) Tyumen State University, Department of Cryosophy, Tyumen, Russia
(7) Michigan State University, Department of Geography, Environment, and Spatial Sciences, East Lansing, Michigan, USA
(8) Northern Michigan University, Department of Earth, Environmental, and Geographical Sciences, Marquette, Michigan, USA
Degradation of permafrost poses a threat to the current and future infrastructure of the north circumpolar region. Rapid warming of the Arctic has led to rising permafrost temperatures and the potential for severe damage to infrastructure. The thermal state of permafrost in the near future must be considered carefully when planning future societal and industrial development of the Arctic.
Our aim was to assess permafrost-thaw related geohazards at an unprecedentedly high spatial resolution (<1 km^2) across the entire circumpolar region for a near–future scenario. The possible consequences for human activity were estimated by quantifying the amount of basic infrastructure at risk (roads, railways, airports, pipelines, buildings, human settlements, and industrial areas). We first performed statistical ensemble modeling of permafrost properties (ground temperature and active-layer thickness) under current conditions. Next, we predicted permafrost conditions around mid-century using climate forcing scenarios that considered different pathways of human-induced greenhouse gas emissions. Finally, we formulated geohazard indices employing the projections of permafrost thaw, changes in active-layer thickness, and soil and terrain properties affecting permafrost stability.
Considering a moderate scenario, around 70% of the current infrastructure exists in areas where near-surface permafrost has high potential for thaw by mid-century. Moreover, one-third of the circumpolar built environment is located in high-hazard areas where thaw-related instability may cause severe damage. Sustainable development of Arctic communities and utilization of natural resources requires that these issues are addressed in planning at regional and local levels. Our modeling improves knowledge about large-scale variability in permafrost-thaw related geohazards and facilitates targeting localized analyses.
Roza Laptander1 & Timo Kumpula2
(1) University of Lapland, Arctic Centre, Rovaniemi, Finland
(2) University of Eastern Finland, Department of Geographical and Historical Studies, Joensuu, Finland
The traditional landuse in the Yamal is generally based on the Nenets reindeer herding. However, the hydrocarbon industry is presently the source of most ecological changes in the Yamal peninsula and socio-economic impacts experienced by nomadic Nenets reindeer herders who move annually between winter pastures at treeline and the coastal summer pastures nearby the Kara Sea.
In the central part of the Yamal peninsula, which is a permafrost area, both natural and anthropogenic changes have occurred during the last 50 years. We have studied gas field’s development and natural changes, like increases in shrub growth, cryogenic landslides, drying lakes in the region and these impacts to the Nenets reindeer herding.
The Nenets with collective and private owned herds of reindeer have proven adapt in responding to a broad range of intensifying industrial impacts, at the same time, as they have been dealing with symptoms of a warming climate and thawing permafrost phenomena.
The results of climate change together with the industrial development of the Yamal Peninsula have a serious impact to the Nenets nomadic reindeer husbandry. Their consequences made the Nenets reindeer herders change their migration routes and the way of working with reindeer. During several years, we were doing interviews with the Nenets reindeer herders about the influence of climate change and industrialization of the tundra on the quality of the Nenets’ life and their work with reindeer. Reindeer herders said that the impacts of the industrial development have reduced their migration opportunities. Due to the concentration of a high number of reindeer in certain areas, nowadays the quality of pastures is quite poor. It has fatal effect during icing on the tundra in the winter. At the same time, in the summer reindeer have more food because of the increasing of green vegetation on the tundra.
Here we detail both the climate change impacts and spatial extent of gas field growth, landslides drying lakes, shrub increase and the dynamic relationship between the Nenets nomads and their rapidly evolving social-ecological system.
Session 2: Approaches to research and adaptation
T-MOSAiC and RATIC: Connections and opportunities
Warwick Vincent1, João Canário2, Donald A. Walker3, Jana Peirce3 & Vladimir Romanovsky4,5
(1) Université Laval, Center for Northern Studies, Québec, Canada
(2) University of Lisbon, Instituto Superior Técnico, Lisbon, Portugal
(3) University of Alaska Fairbanks, Institute of Arctic Biology, and Department of Biology and Wildlife, Fairbanks, Alaska, USA
(4) University of Alaska Fairbanks, Geophysical Institute, Fairbanks, Alaska, USA
(5) Tyumen State University, Department of Cryosophy, Tyumen, Russia
MOSAiC (The Multidisciplinary drifting Observatory for the Study of Arctic Climate) is a multinational year-round study of the central Arctic Ocean to measure the coupling between atmosphere, sea ice, ocean and ecosystem processes. T-MOSAiC (Terrestrial Multidisciplinary distributed Observatories for the Study of Arctic Connections) brings that focus to land. It is an IASC pan-Arctic, terrestrial research program that extends the activities of the flagship MOSAiC program planned for 2019-2020. The objective of the T-MOSAiC satellite program is to coordinate complementary activities that will aid and benefit from MOSAiC by extending the work to the lands surrounding the Arctic Ocean and to the northern communities who live on those lands.
The defining focus of the RATIC initiative on the impacts and interactions of climate and infrastructure in the Arctic provides its own complement to the broad system-level focus of T-MOSAiC with its themes of connectivity, gradients, discontinuities and thresholds, feedbacks, extreme events, legacy effects and emergent properties. RATIC fills in the little “i” in T-MOSAiC through its goal of promoting sustainable Arctic infrastructure as a key research theme requiring collaboration across disciplines and geographic boundaries. RATIC workshops at IASC conferences provide a valuable forum for scientists to discuss research needs and priorities related to both RATIC and T-MOSAiC, to explore opportunities for international multidisciplinary collaboration, and to promote more involvement by early career scientists, local communities, governments, and industry. More information about RATIC is provided at www.geobotany.uaf.edu/ratic, and the Science Plan for T-MOSAiC can be downloaded at: www.tmosaic.com/science-plan.html.
Donald A. Walker1, Amy Breen2, Billy Connor3, Ronnie Daanen4, Lisa Druckenmiller1; Robbin Garber-Slaght5; Jack Hébert5, Ben Jones6, Anja Kade1, Misha Kanevskiy7, Gary Kofinas1, Anna Liljedahl6, Dmitri Nicolsky8, Jana Peirce1, Martha Raynolds1, Vlad Romanovsky8, Yuri Shur7, Warwick Vincent9
(1) University of Alaska Fairbanks, Institute of Arctic Biology, Fairbanks, Alaska, USA
(2) University of Alaska Fairbanks, International Arctic Research Center, Fairbanks, Alaska, USA
(3) University of Alaska Fairbanks, Institute of Northern Engineering, Fairbanks, Alaska, USA
(4) Alaska Department of Geology and Geophysical Surveys, Fairbanks, Alaska, USA
(5) Cold Climate Housing Research Center, Fairbanks, Alaska, USA
(6) University of Alaska Fairbanks, Water and Environmental Research Center, Fairbanks, Alaska, USA
(7) University of Alaska Fairbanks, Institute of Northern Engineering, Fairbanks, Alaska, USA
(8) University of Alaska Fairbanks, Geophysical Institute, Fairbanks, Alaska, USA
(9) Center for Northern Studies, Laval Université Laval, Québec City, Québec, Canada
Ice-rich permafrost is at the center of a web of interacting ecosystem components that we call the IRP system (IRPS). Our ultimate goal is to understand IRPS at local, regional and circumpolar scales. We are particularly interested in how differences in vegetation, water, and time influence the accumulation and degradation of ground ice in IRP landscapes, and how the loss of ground ice can radically change these landscapes, their components, and the infrastructure built on them. The proposed project offers a transformative view that places IRP at the center of change to social-ecological systems in many areas of the new Arctic. Our key questions are: “How are climate change and infrastructure affecting IRPS?”, “What roles do ecosystems play in the development and degradation of IRP?”, and “How can people and their infrastructure adapt to changing IRP systems?"
There is an immediate need to develop more strategic approaches to mitigation and adaptation informed by science and engineering in collaboration with local observations, knowledge, and preferences. Much of the response to permafrost-related damage has been incremental actions driven by the necessity to repair and stabilize existing roads and structures. Our initial focus is at Prudhoe Bay and Point Lay, Alaska, where permafrost temperatures are changing rapidly with large impacts to ecosystems, infrastructure, and communities. Both areas provide excellent examples of IRP-related issues relevant to many other areas of Alaska and the Arctic. We will develop three IRP observatories: 1) Roadside IRP Observatory in the Prudhoe Bay oilfield; 2) Natural IRP Observatory remote from infrastructure; and 3) Village IRP Observatory at Point Lay. The Prudhoe Bay region has the best historical record of geoecological change within the Arctic with key legacy datasets and good collaboration between industry and science. We will revisit permanent plots and remap Prudhoe Bay vegetation and landscapes first studied in the 1970s.
Point Lay has received less research and agency attention than other climate-impacted communities, yet its thaw related issues are among the most severe. The Cold Climate Housing Research Center will work with the Regional Housing Authority, community residents, local high school students and regional planners to collaboratively produce adaptive housing strategies. A permafrost and infrastructure symposium hosted by the community will bring together US-Canadian science and engineering expertise to discuss a range of public infrastructure issues relevant to many Arctic villages. Our team’s work with the Alaska Department of Transportation will advance knowledge on IRP-related impacts to roads and industrial infrastructure and contribute to best practice guidelines for road and airport construction. Science education and training components will reach K-12, undergraduate, graduate, and post-doctoral students.
Robbin Garber-Slaght, PE1
(1) Cold Climate Housing Research Center, Fairbanks, Alaska
Alaska contains some 300 Native villages scattered across 360 million acres ranging from temperate rainforests in the southwest to Arctic tundra in the north. Most are only accessible by airplane. There are few services and most of the food, fuel, and supplies are brought in by barge (in the summer) or plane. Alaska’s coastal communities are feeling the brunt of climate change today.
The community of Newtok on the southwest coast has been battered by a changing climate for over 15 years. Because of melting sea ice that used to protect their shore, the village regularly floods during the fall storm season. They have lost their sewage loon and landfill to flooding, and every year they lose about 24 m of coastline. The closest homes are within five meters of the edge. Like many Alaska Native communities, Newtok was never a permanent settlement. But because permanent infrastructure was built there by outside agencies, it became permanent which has led to many of the sustainability problems we are seeing today.
CCHRC is working with the community of Newtok to provide housing that is more sustainable than what they currently have, in a location that is better suited for permanent settlement. This presentation will highlight CCHRC’s work with rural Alaskan communities to develop a process for locally driven sustainable housing that is being used as part of the Newtok relocation.
Dmitrii Sergeev & Irina Utkina1
(1) Sergeev Institute of Environmental Geoscience, Russian Academy of Sciences, Moscow, Russia
The long-range linear structures are under influence of local permafrost diversity of various landscapes as well under the different microclimate variabilities. An example of the features of the evolution of the natural-technical system of a railway embankment under the conditions of the Vorkuta tundra demonstrates the complexity of this task.
A possible (although time-consuming) solution is to develop individual forecasts for typical combinations of landscapes and engineering structures. Such forecasts need special discussion about input data preparation rules. Possible sources of models’ input are geological surveys (to clarify the local landscape features), regional GTN-P data from the monitoring of the undisturbed permafrost conditions (for calibrating the regional models of permafrost response to climate change) and regional climate models (for specifying the climate impact scenarios).
To ensure the comparability of the results of permafrost forecasting, a transparent description of the assumptions and setting boundary conditions is needed. In particular, the expected lifetime of the infrastructure affects the depth of research and the characteristics of the model. Forecasts with a duration of more than 50 years should be provided with data on direct downhole measurements with a depth of at least 300 m. It’s important for setting the correct initial temperature field configuration.
It is recommended to use the various key indicators of the state and dynamics of permafrost, which are correlated with the specifics of making management decisions. It’s recommended to use the indicator of the maximal depth of interannual temperature fluctuations to determine the depth of surveys and monitoring. It’s recommended to the annual average integral fraction of liquid moisture in a ten-meter layer of soil to estimate the bearing capacity of the ground soils, and the depth of permafrost table. They are important information for designers.
Peter Schweitzer, Gertrude Saxinger, Olga Povoroznyuk1
(1) University of Vienna, Austrian Polar Research Institute, Vienna, Austria
Infrastructure, while planned and built by humans, has in turn significant impacts on people’s lives. Arctic infrastructure development often has even bigger impacts than similar projects in temperate zones, as it affects fragile ecosystems and remote communities. Social scientists have started to study infrastructure later than engineers but can bring important perspectives to the field. By engaging concepts such as “infrastructural violence”, “enclaves” and “uneven development”, Arctic social scientists attempt to understand the socio-political conditions, problems and benefits of infrastructure development in remote regions.
Arctic marine shipping (AMS) is projected to increase significantly due to shrinking sea ice and increased resource extraction activities. This requires the construction of new port and subsidiary facilities, which will further transform the natural, built and social environments of the High North. The so-called Northern Sea Route (NSR) is the most productive passageway for AMS. Current and planned research – that combines ethnographic methods with the analysis of satellite images will allow to quantify growth and decline – will be introduced.
Corporate Social Responsibility (CSR) activities are becoming increasingly important practices in infrastructural (mega) projects. However, not all CSR types and ways of their implementation are beneficial, and some become issues of dispute between project proponents, locals and political actors. This section will address the nexus of social impact assessment, CSR and the social license and will raise further critical issues in the context of infrastructural development in the Arctic.
Poster Session
Arctic infrastructure at high risk by 2050
Olli Karjalainen1
(1) University of Oulu, Geography Research Unit, Oulu, Finland
It is becoming ever more evident that perennially frozen ground, permafrost, is warming at a global scale. In addition to the impacts on ecosystems, hydrology and geomorphology of permafrost environments, degradation of permafrost involves serious societal aspects. One of these is a threat to man-made engineering structures posed by thaw of ice-rich permafrost and resulting potential for ground subsidence, mass movements, and loss of structural bearing capacity.
We used statistical ensemble modelling to assess the temperature and extent of current and future near-surface permafrost, and to project permafrost thaw-related geohazards potentially yielding damage to infrastructure. Our approach combined an Arctic-wide extent (land areas north of 30°N) and a high spatial resolution (~1 km), which allowed for performing the first quantitative assessment of infrastructure elements (e.g., transportation and industrial constructions) and human settlements at risk in the Northern Hemisphere.
We found that nearly four million people and around 70% of the current infrastructure in the Northern Hemisphere locate on potentially thawing near-surface permafrost by midcentury. Further analyses of the geotechnical hazard potential of permafrost thaw revealed that about one-third of all infrastructure is in high-risk areas. This includes 45% of the hydrocarbon extraction fields in the Russian Arctic and long segments of oil and gas pipelines. Alarmingly, we found that achieving the targets of the Paris Agreement would not do much to reduce the hazard potential by midcentury.
Our results provide important insights into the broad-scale development of geohazards in the near future and allow for identifying high-risk regions where localized hazard assessments should be targeted to facilitate sustainable development of Arctic communities. Thereby, our findings contribute to successful management of the impacts of the ongoing climate change.
Kelcy Kent & Howard Epstein1
1 University of Virginia, Department of Environmental Science, Charlottesville, Virginia, USA
Nitrogen availability strongly influences plant productivity and distribution in Arctic environments. Recently, the Arctic has experienced warmer temperatures and the resulting permafrost thaw along the coastal Arctic tundra has caused ground subsidence, ponding, and the release of solutes and nutrients, impacting vegetation distribution. In patterned ground (polygon) permafrost systems, nutrient cycling can vary substantially across ice-wedge polygon successional stages. A better understanding of fine-scale spatial and temporal nutrient cycling among these ice-wedge polygon trajectories will improve the ability to predict tundra response to warming. This study took place during July and August of 2018 in a coastal tussock tundra site in Jago, Alaska. The study aims to identify and quantify plant-available inorganic nitrogen and total dissolved nitrogen in the soil, water tracks, and ponds of various successional stages of ice-wedge polygons, and to quantify %N and C:N ratios in plant biomass in each successional stage. Water samples were taken from various inundated or water-logged ice-wedge polygon successional stages and water tracks flowing throughout the transect, and NO3- and NH4+ uptake in each site type were assessed with buried bag experiments (still in progress) and AgWestern ion probes. Biomass was clipped from each successional stage to compare functional group composition, mass, and C:N ratios (still in progress). Clippings of the common wet tundra sedge Eriophorum angustifolium was also taken from each successional stage to use as a benchmark species to compare C:N ratios across site types. Preliminary results show that though there are relatively small concentrations of NH4+, and especially NO3-, at all sites sampled, there is a greater availability of NH4+ and TDN in sites that are experiencing some degree of degradation and inundation. A one-way ANOVA found significant differences in NH4 (p= 0.0046), TDN (p=0.00026), and DON (p=0.0002; data not pictured) among sites. AgWestern soil ion probes suggest greater N uptake at sites that have experienced some degree of degradation, and %N by weight of plant tissue from E. angustifolium increases slightly across successional sites. Greater average C:N ratios in E. angustifolium are found in tussock tundra (polygon center) and undegraded ice-wedge polygon sites. This preliminary data suggests that when permafrost thaws, the soil organic matter and minerals within the permafrost become available for remobilization and uptake, and the resulting increase in water may play a key role in the storage and transport of nutrients, influencing vegetation response to the warming Arctic. Future work includes completing the assessment of the buried bag experiments to compare with the data from the AgWestern ion probes; analysis of biomass clippings from each ice wedge polygon successional stage for vegetation functional group composition, mass, and C:N ratios; and further studies of nutrient availability in relation to ice-wedge degradation or stabilization and the impending effect on Arctic tundra vegetation in additional sites in the coastal Arctic tundra.
Peter Schweitzer, Alexis Sancho Reinoso, Gertraud Illmeier, Olga Povoroznyuk, Gertrude Saxinger, Sigrid Schiesser1, Natalia Krasnoshtanova, Vera Kuklina2
(1) University of Vienna, Department for Social and Cultural Anthropology
(2) Sochava Institute for Geography, Russian Academy of Sciences, Irkutsk
The Arctic and Subarctic have gained a surprising amount of attention in recent years. What used to be the ‘remote’ backwaters of global economic and political currents have morphed into a new frontier of geopolitics, resource extraction, and developmental designs. New transportation infrastructure often plays a critical role in these transformations. But its effects – accessibility, the shrinking of social and physical distance, the increased speed of connection – are not uncontested. On the one hand, those for whom ‘remoteness’ has been an asset, are often among the opponents of such developments. New transportation infrastructures are often not built to make the lives of local residents easier but to move cargo. Thus, there are ‘winners’ and ‘losers’ of such infrastructural developments.
Our key research question: Given the technosocial entanglement of people and infrastructure, how do changes in remote transportation systems affect human sociality and mobility?
CoRe is located in North Asia, at the junction of eastern Siberia and the Russian Far East. We call the area the BAM region because it is defined by the Baykal-Amur Mainline (BAM) railroad and its sidetracks. In that region there are pockets with a long history of industrial development and resource extraction, while many parts of the area have been little affected by Soviet and post-Soviet modernization efforts. Current attempts to revitalize, improve and extend the railway network serve as the backdrop for our project.
Rustam Khairullin, Artem Khomutov, Marina Leibman1
(1) Earth Cryosphere Institute, Tyumen Scientific Centre, SB Russian Academy of Sciences, Tyumen, Russia
Manual processing and visual interpretation were implied to very high-resolution data (Qiuckbird, Geoeye satellites). Various types of anthropogenic landscapes are easily identifiable, such as geocryological relief forms within Vaskiny Dachi research station. Using this precise data, “Object density” maps of anthropogenic areas and thermocirques were created for years 2009 and 2013. After this, density growth rate was estimated and spatial correlation between changes in infrastructure and thermocirques was calculated. Correlation type is weak positive and equals 0.15.
Middle resolution data (Landsat, Sentinel-2) are more suitable for studying Earth’s surface through complex of vegetation indexes. For this purpose, Landsat database was created for Ob’ Bay region and automatic querying and processing algorithm was developed. Algorithm includes a selection of scenes depending on date, spatial intersections and vegetation development rate through air temperature (phenology). Algorithm calculates NDVI, NDWI and SWVI and compares them between 2 scenes.
On the other hand, Sentinel-2 data was used to examine relatively new object such as gas emission craters (GEC). Hypothesis of GEC formation allowed to develop prediction maps based on known triggers and controls interpreted on imagery by supervised classification.
In addition, UAV surveying was used as a compromise between field methods of monitoring and classical remote sensing. UAV survey provides information about landscapes (mostly vegetation patterns and DEM) through 3D models or orthophotomaps with resolution up to 0,02 m/pix. UAV survey was applied to old and new research objects: thermocirque edges in 2017 and 2018 were compared to precisely detect the rate of retreat. One more object, peat plateau near Gaz-Sale, was surveyed by UAV as well and orthophotomaps were compiled. At the same time, tacheometric survey was made to complement UAV survey. Orthophotomaps appeared to be efficient and precise. Very high-resolution satellite imagery analysis established nonlinearity of peatland degradation process and destruction of inter-polygonal depressions.
Elena A. Babkina1, Marina O. Leibman1, Artem V. Khomutov1, Damir R. Mullanurov1, Anatoly A. Gubarkov2
(1) Earth Cryosphere Institute, Tyumen Scientific Centre, SB Russian Academy of Sciences, Tyumen, Russia
(2) Tyumen Industrial University, Tyumen, Russia
The long-term observation series in Central Yamal (since 1993) allows revealing the regularities of active layer depth and ground temperature dynamics under recent climatic fluctuations. Air temperature rise in summer and the increase of summer total precipitation in 2012 and 2016 caused a significant active layer deepening in all types of surface as compared with the previous period of 1993-2011 (12-20% in 2012, 24-37% in 2016). The ground temperature rise also observed through entire of all the surface types of the active layer during the warmest 2012 and 2016. And at 10 meters depth for 5 years of observations there is a constant increase in the average annual ground temperature (its growth amounted 0,5 °C).
The observations series on the north of Gydan Peninsula and the Pur-Taz interfluve are short (since 2016). Therefore, the only limited conclusion that can be suggested is that lower ground temperatures and less active layer depths correspond to colder climatic conditions.
To assess the impact of all kinds of technogenic activities, monitoring of active layer depth was initiated across vehicle tracks and in rather a recent sand quarry. An increase of active layer depth within the areas with technogenic impact in comparison with natural conditions was revealed to be 10-20%. On the disturbed surface of the quarry the melting of the ice wedges continues. Portions of quarry where vegetation cover restores active layer depths tend to values characteristic of natural conditions.
Observations in Central Yamal also include measurements of seasonal subsidence (since 2007).
Subsidence at Vaskiny Dachi CALM grid ranges between 0 and 70 mm depending on soil moisture, snow thickness and soil salinity. More moisture and more snow show maximum subsidence. Saline soils show subsidence 25-35% less than non-saline. Salinity acts directly, through increase in unfrozen water content and thus less ice in winter and less subsidence in summer. Moisture content and snow cover provide moisture for active-layer ice lenses in winter, at the same time, determining slower freezing in winter and more intensive frost-heaving. More heaving in cold season, deeper subsidence in warm season.
Agapkin Ivan2, Kal'bergenov Roman1, Karpenko Fedor1
(1) Sergeev Institute of Environmental Geoscience of Russia Academy of Science, Moscow, Russia
(2) Vernadsky Institute of Geochemistry and Analytical Chemistry, Russia Academy of Science, Moscow, Russia
Throughout the existence of the Earth, cyclical climate changes took place: glacial epochs were replaced by interglacial periods. Today, climate warming is abnormally fast, one of the theories explaining global warming is the greenhouse effect.
In the arctic regions, an increase in temperature is particularly dangerous due to an increase in, or even thawing, permafrost temperature. The temperature change of permafrost soils affects their structure and as a result their mechanical properties.
This paper presents results of the research of the temperature influence on the strength properties of frozen soils of the area near r. Yuribey (middle Yamal). Strength properties (cohesion) determined by triaxial shear test at temperatures from -1 to -8 ° С.
When soil freezes cryogenic concentration occurs as a result of which salinity increases from top to bottom along the section from 0.05 to 1%. Along with increasing salinity down the section decreases the magnitude of cohesion. As a result of cryogenic concentration at a depth of 20-23 m, cryopeg formed, soils in the cryopeg zone have salinity of 1%, and the minimum cohesion value appears at a temperature of -3 ° C.
Research results show patterns of cohesion change with increasing temperature and increasing salinity in loam and sand. In the sands with an increase in salinity from 0.01 to 0.05, the cohesion decreases by half at a temperature of -1 ° C. Loams with a salinity of 0.5% have minimal cohesion at 0.17 MPa at a temperature of -2 ° C, and when the temperature rises -1 ° C almost no clutch.
Thus, the work evaluates the effect of temperature and salinity on the cohesion values of soils in the study area r. Yuribey. The important point is that frozen soils can significantly lose their strength without going into a thawed state.
The obtained data will be used for further experimental studies of the influence of changes in climatic and geocryological conditions on the properties of the permafrost soils of the Arctic zone.
Dmitrii Sergeev & Irina Utkina1
1 Sergeev Institute of Environmental Geoscience, Russian Academy of Sciences, Moscow, Russia
Permafrost responds to climate change. This creates unforeseen problems for designers, who are forced to provide special design solutions for future structures. On the other hand, this creates problems for existing facilities. There will be a high risk of the situation when the rising infrastructure finds itself in a situation that there will not be enough money to repair it or create the engineering protection.
It is necessary to use the correct indicators that will help investors, economists and design engineers to make the adequate decisions on the base of modeling and permafrost forecasting. The indicators used now, such as the mean annual temperature of the grounds at the depth of penetration of seasonal fluctuations, the depth of seasonal thawing and the characteristics of the areal distribution of permafrost, are of little use in management decision-making practice. These indicators do not reveal all the features of the state and dynamics of permafrost. For example, the salinity of soils leads to a significant loss of their bearing capacity with increasing temperature of the frozen soil, although it remains formally frozen in negative temperature interval. Another example is the depth of penetration of seasonal temperature fluctuations, which dramatically decreases when the permafrost starts to thaw.
Thus, a methodical approach is required which would help to form the expanded list of regional indicators of the state and dynamics of permafrost. Such indicators should be objective, comparable and relatively easy to determine in the field. For example, the indicator “position of the southern boundary of permafrost” is difficult to determine, since it strongly depends on the scale of mapping and it is difficult to assure by the field observation.
The experience of work in the North of the European part of Russia and in Transbaikalia allowed developing a generalized approach to the compilation of regionally significant indicators of the state of permafrost. This approach provides in addition to standard types of analysis: 1) justification of areas with constantly thawed, partially frozen and constantly frozen conditions, 2) mapping of areas with different ice content, gas content and salinity of grounds, 3) fixation of representative areas which the depth of seasonal fluctuations of temperature has the tendency to change.
In planning the geocryological forecast it is necessary to take into account: 1) the inhomogeneity of the permafrost table position and the case of phase transition zone is deeper than the penetration of seasonal temperature fluctuations, 2) the presence of fluids that carry heat in the permafrost thickness, 3) the presence of the instable temperature field associated with the heat exchange history, 4) the presence of saline or gas-containing rocks in permafrost.
All these considerations can be summarized in the form of a list of indicators of the state of permafrost conditions applicable both for each source of field data (pit, borehole), and for larger zoning taxons. The list includes such indicators as the depth interval within the seasonal temperature fluctuations are observed; averaged air, surface and soil temperatures at different depths, averaged within a group of sites (related to the coldest and warmest landscape types), as well as areas with different ground ice content, depth intervals with partial freezing and thawing (with the establishment of a criterion for unfrozen water), the temperature of the onset of phase transitions at the bottom of the active layer, the sign and magnitude of the geothermal gradient below the depth of penetration of seasonal temperature fluctuations.
For these indicators it is recommended to build the special maps of the state and dynamics of the geocryological conditions that is important for economic development of the territory.
Evgenii.M. Babkin1, Artem V. Khomutov1, Elena A. Babkina1, Anatoly A. Gubarkov2, Yury A. Dvornikov1, Dmitry A. Kaverin3, Rustam R. Khairullin1, Marat R. Sadurtdinov1, Maria S. Sudakova1
(1) Earth Cryosphere Institute, Tyumen Scientific Centre, SB Russian Academy of Sciences, Tyumen, Russia
(2) Tyumen industrial University, Tyumen, Russia
(3) Institute of Biology, Komi Scientific Centre, UB Russian Academy of Sciences, Syktyvkar, Russia
Ice wedge polygonal systems of peatlands were studied in the North-Eastern part of the Pur-Taz interfluve in the north of West Siberia.
The combination of very high-resolution satellite images with even more detailed UAV orthophotomaps was used to assess changes in the surface of peatlands. The analysis of multi-temporal satellite images showed that the changes that occurred for more than 10 years from 2005 to 2016 are comparable with the changes for the last 2 years from 2016 to 2018.
Ongoing monitoring of permafrost parameters showed that climatic fluctuations of 2012-2013 caused thawing of ice wedges at different rates depending on variability of environmental factors and degree of technogenic influence. Extremely warm period conditions of 2016 contributed to the increased ice wedge thawing.
Ground-penetrating radar (GPR) studies of experimental and methodological nature allowed investigating different aspects. Spatial variability of seasonal thawing depth and the configuration of the top of the permafrost in the cross-section, the impact on them of natural and technogenic factors were studied in detail using this method. GPR also enabled the development of basic principles of integrated study of the top layer of the permafrost and the relief of polygonal peatlands with ice wedges in the cross-section and their dynamics in time.
The new method for the study of aeolian sedimentation in the peat areas was proposed and tested. The decrease in the layer of aeolian deposits with the distance from the source of deflation (road embankment) occurs quite sharply. The process is described by a degree equation with decent approximation R2 = 0.82 for natural processes.
Based on all conducted field and laboratory studies and remote sensing data we defined certain general patterns. They imply the influence of last year’s climatic changes in combination with technogenic factors on dynamics of peatland polygonal ice wedge systems in the study area. As a result, it was possible to make an assessment map of the degradation degree of peatland ice wedges. Peatlands are classified according to the degree of potential degradation of the surface and the top layer of permafrost.
Valery I. Grebenets & Vasily A. Tolmanov1
(1) Lomonosov Moscow State University, Department of Cryolithology and Glaciology
The Arctic is the territory of perspective development. Strengthening of antropogenic influence and noticeable climatic changes affecting the state of permafrost are negatively manifested for linear technogenic systems (LTS). We conducted complex field studies, numerical modelling, forecasting of the LTS state under changing conditions in the Arctic regions and defined five main types of LTS: pipelines (aboveground, onground and underground), city waterlines, electric lines, autoroads and railways. Pipelines, paved on the permafrost are traditionally arranged on supports that are raised above the surface and frozen into the ground; the main problem in this case is uneven frost heaving in the active layer. Deformations associated with dangerous cryogenic processes - thermokarst and thermoerosion develop in the zone of discontinuous and sporadic permafrost, which intensity is increasing due to climatic changes of recent decades; revealed that 30-40% of such LTSs are substantially deformed or even destroyed after 5-10 years of operation. About 70% of underground communications in the largest Arctic cities of Russia are in poor condition. We have analysed the potential hazards for the first time for all regions of Eastern Siberia and the Far East (about 300 administrative areas: uluuses, kujuuns) for roads and railways associated with thermokarst, thermoerosion, thermoabrasion, icing, frost heaving, frost cracking, moving of the rock glaciers. Negative effects from dangerous cryogenic processes are manifested in the form of frost cracks, formation of ice, dips, sliding slopes, wavy deformations, strengthening thixotropy of soils, lowering the bearing capacity of frozen bases.
Fieldwork was supported by the grant. RFBR 18-05-60080 “Dangerous nivalglacial and cryogenic processes and their impact on infrastructure in the Arctic”. Mapping and risk calculation performed within support of the grant of the Russian Geographical Society No. 15/17: “Current state and dynamics of hazardous natural processes affecting the existing and prospective transport network of Siberia and the Far East.”
Donald A. Walker1, Howard E. Epstein2, Jozef Šibík3, Uma Bhatt4, Vladimir E. Romanovsky5, Amy L. Breen5, Silvia Chasnikova‑3, Ronald Daanen6, Lisa A. Druckenmiller1, Ksenia Ermokhina7, Bruce C. Forbes8, Gerald V. Frost9, Jozsef Geml10, Elina Kaärlejarvi11, Olga Khitun12, Artem Khomutov7,13, Timo Kumpula14, Patrick Kuss15, Marina Leibman7, Georgy Matyshak16, Natalya Moskalenko7, Pavel Orekhov7, Jana Peirce1, Martha K. Raynolds1, & Ina Timling1
(1) University of Alaska Fairbanks, Institute of Arctic Biology & Department of Biology & Wildlife, Fairbanks, Alaska, USA
(2) University of Virginia, Department of Environmental Sciences, Charlottesville, Virginia, USA
(3) Slovak Academy of Sciences, Institute of Botany, Plant Science and Biodiversity Center, Bratislava, Slovak Republic
(4) University of Alaska Fairbanks, Geophysical Institute & Department of Atmospheric Science, Fairbanks, Alaska, USA
(5) University of Alaska Fairbanks, International Arctic Research Center, Fairbanks, Alaska, USA
(6) State of Alaska, Division of Geological & Geophysical Surveys, Fairbanks, Alaska, USA
(7) Russian Academy of Sciences – Siberian Branch, Tyumen Scientific Center, Earth Cryosphere Institute, Russia
(8) University of Lapland, Arctic Center, Rovaniemi, Finland
(9) Alaska Biological Research, Inc., Fairbanks, Alaska, USA
(10) Naturalis Biodiversity Center, The Netherlands
(11) Umeå University, Department of Ecology and Environmental Sciences, Umeå, Sweden
(12) Russian Academy of Sciences, Komarov Botanical Institute, St. Petersburg, Russia
(13) University of Tyumen, Tyumen, Russia
(14) University of Eastern Finland, Joensuu, Finland
(15) University of Zürich, Institute of Systematic and Evolutionary Botany, Zürich, Switzerland
(16) Lomonosov Moscow State University, Department of Soil Science, Moscow, Russia